Chemical Bonding and Molecular Structure – Complete Guide For Class 11 Chemistry Chapter 4
Welcome to iPrep, your Learning Super App. Our learning resources for Chapter 4, “Chemical Bonding and Molecular Structure,” in Class 11 Chemistry are meticulously designed to ensure students gain a comprehensive understanding of this essential topic. These resources include detailed notes on the different types of chemical bonds, such as ionic, covalent, and hydrogen bonds, as well as their formation and properties. Additionally, we provide in-depth explanations of molecular geometry and the VSEPR theory, alongside diagrams that illustrate molecular shapes and bond angles. The study materials also cover hybridization and molecular orbital theory, helping students grasp complex bonding concepts. Finally, interactive quizzes and practice problems are available to reinforce learning and test comprehension.
What Is Chemical Bonding And Molecular Structure?
Chemical Bonding and Molecular Structure refers to how atoms come together to form molecules and compounds. It involves understanding the forces that hold atoms together in various bond types, such as ionic, covalent, and metallic bonds. This chapter delves into how these bonds form, the arrangement of electrons, and the resulting molecular shapes and structures. Understanding these concepts is crucial for grasping how substances interact and react at a molecular level.
This chapter emphasizes the significance of different types of bonds, such as ionic, covalent, and hydrogen bonds, and their role in maintaining the structure and function of biomolecules. Students learn about molecular geometry, which influences the three-dimensional shapes of molecules, crucial for understanding biochemical reactions. The chapter also discusses the concept of hybridization, explaining how atomic orbitals mix to form new hybrid orbitals, affecting molecular shape and bond formation. Furthermore, the study of molecular orbitals and resonance highlights the stability and reactivity of molecules in biological systems.
Lewis Electron Dot Structure and the Octet Rule
Further in chemical bonding and molecular structures, we’ll discuss Lewis electron dot structure and the octet rule. This includes-
Lewis Symbols
Lewis symbols are a way to represent the number of valence electrons in an atom. The number of valence electrons corresponds to the group number in the periodic table. Here are some examples:
For example, sodium (Na) has an electron configuration of 1s2,2s2,2p6,3s11s^2, 2s^2, 2p^6, 3s^11s2,2s2,2p6,3s1, which can be simplified to [Ne]3s1[Ne] 3s^1[Ne]3s1. The Lewis structure for sodium is simply “Na” with a single dot representing the one valence electron.
Octet Rule
Atoms strive to achieve the stable electron configuration of the nearest noble gas. This is achieved when an atom has eight electrons in its valence shell, known as the octet rule.
Noble gases like neon (Ne) and argon (Ar) have stable electron configurations:
Atoms will gain, lose, or share electrons to fulfill the octet rule.
Types of Chemical Bonding
There are various types of chemical bonding discussed in the chapter “Chemical Bonding and Molecular Structure”. These include-
Ionic Bonding
Ionic bonding involves electrostatic forces between ions, typically between a metal cation and a non-metal anion. For example, when sodium (Na) and fluorine (F) form an ionic bond, sodium loses an electron and fluorine gains an electron achieving the electron configuration of neon.
Covalent Bonding
Covalent bonding occurs when two atoms, usually non-metals, share electrons to form molecules. This bond type also adheres to the octet rule, where each atom in the bond shares enough electrons to complete its outer shell.
Examples of Covalent Bonds:
- Hydrogen molecule (H₂): Two hydrogen atoms share electrons to achieve the electron configuration of helium.
- Chlorine molecule (Cl₂): Two chlorine atoms share electrons to achieve the electron configuration of argon.
- Nitrogen molecule (N₂): Two nitrogen atoms form a triple bond to achieve the electron configuration of neon.
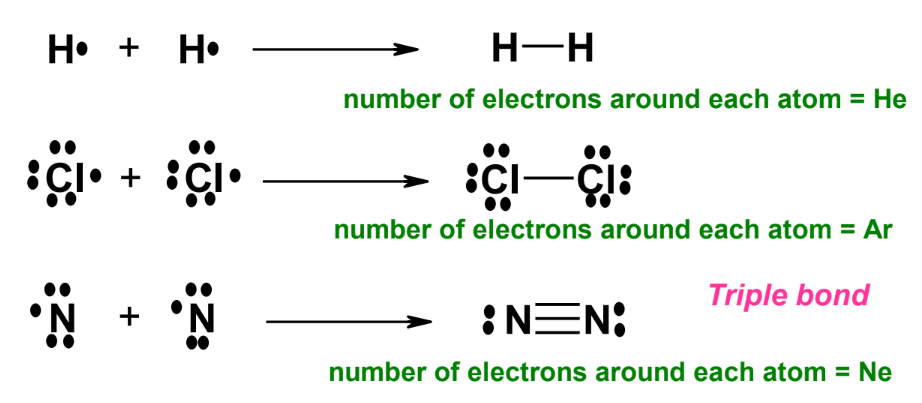
Covalent Bonding in Carbon Compounds
Carbon, with four valence electrons, forms four covalent bonds to achieve a stable octet. In methane (CH₄), carbon forms four single bonds with hydrogen atoms. This sharing results in a stable molecule with eight electrons around carbon, resembling the electron configuration of neon.
Rules for Drawing Lewis Structures
The chapter Chemical Bonding and Molecular Structure clearly states the rules for drawing lewis structure. These include-
- Sum the number of valence electrons from each atom.
- Identify the central atom, usually the one written first in the formula.
- Complete the octets of atoms bonded to the central atom. Hydrogen can only have two electrons.
- Place any leftover electrons on the central atom, even if it results in more than an octet.
- Form multiple bonds if there aren’t enough electrons to complete the octet of the central atom.
Examples:
- Phosphorus trichloride (PCl₃):
- Total valence electrons = 5 + (3 × 7) = 26
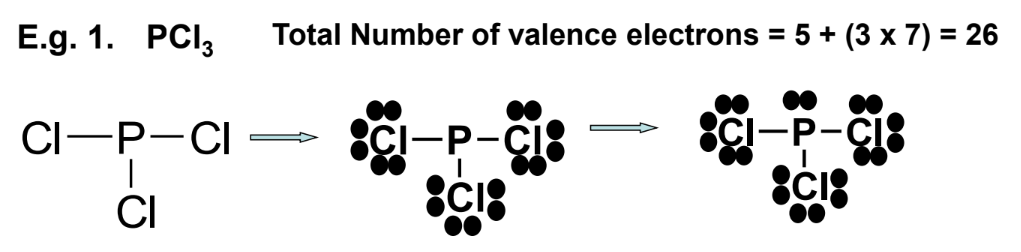
- Bromoform (CHBr₃):
- Total valence electrons = 4 + 1 + (3 × 7) = 26
Exceptions to the Octet Rule in Covalent Bonding
There are various exceptions to the octet rule in covalent bonding as stated in the chapter – Chemical Bonding and Molecular Structure. These include-
1. Molecules with an Odd Number of Electrons
- Nitric oxide (NO): Has 11 valence electrons.
- Nitrogen dioxide (NO₂): Has 17 valence electrons.
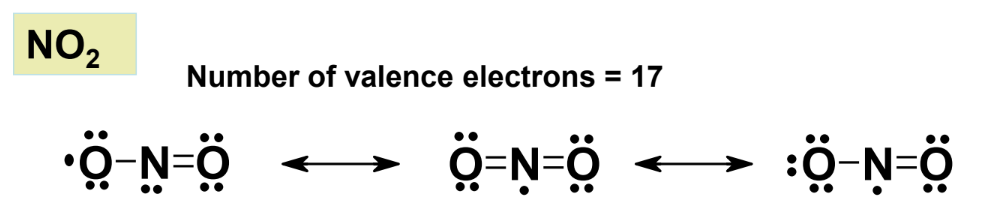
These molecules are known as radicals.
2. Molecules with Less than an Octet
- Boron trichloride (BCl₃): Boron only has six electrons around it. However, it readily accepts a pair of electrons from Lewis bases to establish a stable octet.
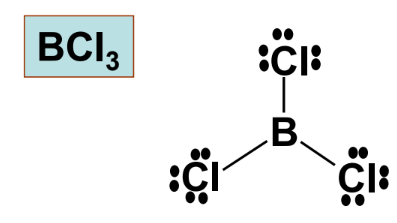
3. Molecules with More than an Octet
Elements from the third period and beyond can exceed the octet rule by using available d-orbitals in bonding. For example:
- Phosphorus pentachloride (PCl₅) has 10 electrons around phosphorus.
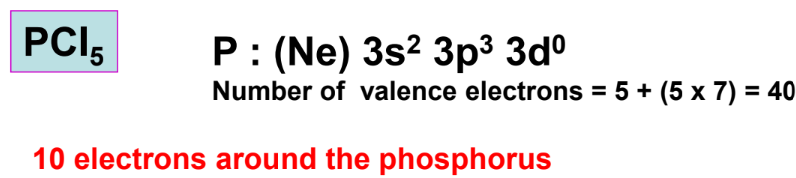
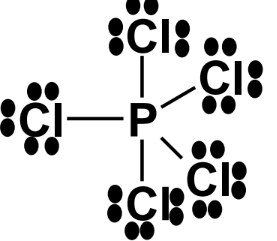
- Sulfur hexafluoride (SF₆) has 12 electrons around sulfur.
Electronegativity and Bond Types
Next in the chapter Chemical Bonding and Molecular Structure, we’ll discuss electronegativity and bond types.
Electronegativity
Electronegativity is the ability of an atom in a molecule to attract electrons to itself. It is influenced by the atom’s ionization energy and electron affinity. The Pauling scale is used to measure electronegativity.
Bond Types Based on Electronegativity Difference
- Non-Polar Covalent: Electronegativity difference < 0.5.
- Polar Covalent: Electronegativity difference between 0.5 and 2.0.
- Ionic: Electronegativity difference ≥ 2.0.
Examples:
- F-F: Electronegativity difference = 0 (non-polar covalent)
- H-F: Electronegativity difference = 1.9 (polar covalent)
- LiF: Electronegativity difference = 3.0 (ionic)
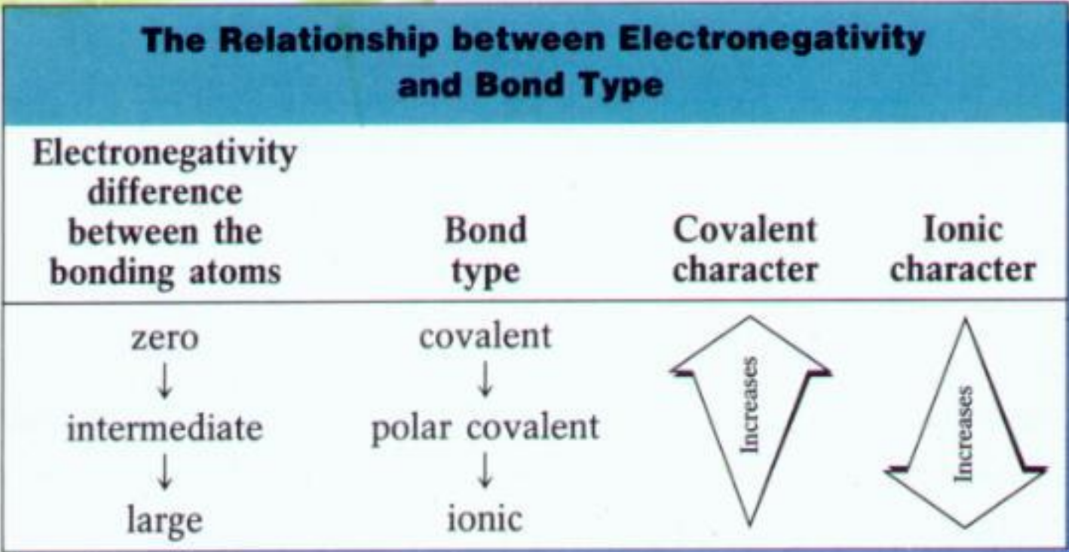
Dipole Moments
As mentioned in the chapter Chemical Bonding and Molecular Structure, Dipole moments occur in polar covalent bonds due to unequal sharing of electrons. For example, HCl has a dipole moment due to the difference in electronegativity between hydrogen and chlorine.
Calculation of Dipole Moment
The dipole moment (µ) is calculated as:
μ=Q×r\mu = Q \times rμ=Q×r
Where QQQ is the charge and rrr is the distance between charges.
Molecular Geometry: Valence Shell Electron Pair Repulsion (VSEPR) Theory
Going forward in the chapter Chemical Bonding and Molecular Structure, we’ll discuss the VSEPR theory.
Introduction to VSEPR Theory
VSEPR theory is used to predict the geometry of molecules based on the repulsions between electron pairs in the valence shell of the central atom.
Steps in Predicting Molecular Geometry:
- Determine the central atom.
- Draw the electron dot structure.
- Find the arrangement of electron pairs.
- Find the arrangement of bonding pairs.
- Determine the geometry based on bonding pairs.
Common Molecular Geometries:
- Linear: Molecules with two electron pairs (e.g., BeH₂).
- Trigonal Planar: Molecules with three electron pairs (e.g., BF₃).
- Tetrahedral: Molecules with four electron pairs (e.g., CH₄).
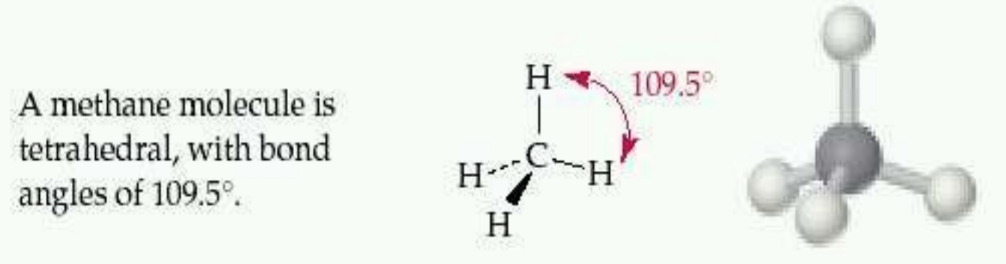
- Trigonal Bipyramidal: Molecules with five electron pairs (e.g., PCl₅).
- Octahedral: Molecules with six electron pairs (e.g., SF₆).
Effect of Lone Pairs on Molecular Geometry
Lone pairs occupy more space than bonding pairs, causing distortions in bond angles. For example:
- Water (H₂O) has a bent shape due to two lone pairs of oxygen.
- Ammonia (NH₃) has a trigonal pyramidal shape due to one lone pair of nitrogen.
Valence Bond Theory (VBT)
Valence Bond Theory is one of the earliest models discussed in the chapter Chemical Bonding and Molecular Structure. It was developed to explain chemical bonding. According to VBT, a covalent bond forms when the orbitals of two atoms overlap, allowing their valence electrons to pair up. This overlap results in a region of high electron density between the nuclei of the two atoms, which holds them together.
Types of Covalent Bonds
Covalent bonds can be classified based on the extent of orbital overlap:
- Sigma (σ) Bond: Formed by the head-on overlap of atomic orbitals, resulting in a bond along the internuclear axis.
- Pi (π) Bond: Formed by the side-to-side overlap of atomic orbitals, resulting in a bond above and below the internuclear axis.
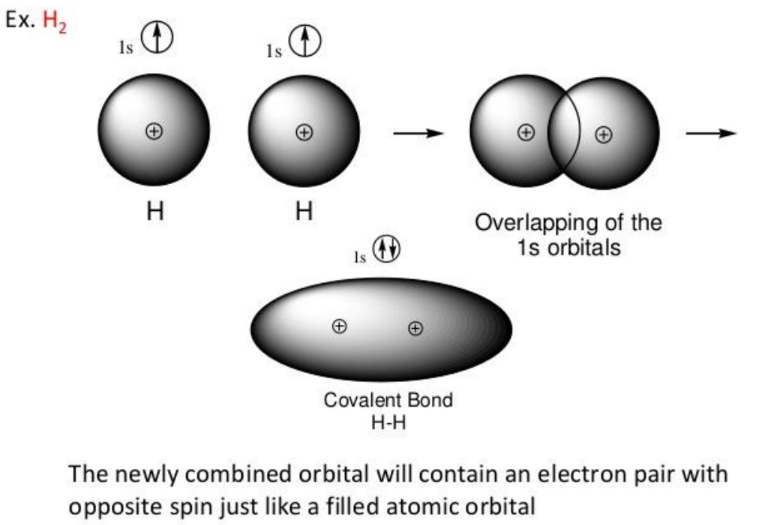
Difference Between Sigma and Pi Bonds
Property | Sigma (σ) Bond | Pi (π) Bond |
Formation | Head-on overlap of orbitals | Side-to-side overlap of orbitals |
Bond Strength | Generally stronger due to greater overlap | Generally weaker due to lesser overlap |
Electron Density | Concentrated along the internuclear axis | Concentrated above and below the internuclear axis |
Rotation around Bond | Allows for free rotation of bonded atoms | Restricts rotation due to the parallel orientation |
Hybridization
Hybridization is the process of mixing atomic orbitals to form new hybrid orbitals that are identical in energy and shape. This concept helps explain the geometry of molecular structures that cannot be accounted for by simple orbital overlap.
Types of Hybridization
- sp Hybridization: Involves the mixing of one s orbital and one p orbital, resulting in two sp hybrid orbitals. The bond angle is 180°, forming a linear geometry.
- sp2 Hybridization: Involves the mixing of one s orbital and two p orbitals, resulting in three sp2 hybrid orbitals. The bond angle is 120°, forming a trigonal planar geometry.
- sp3 Hybridization: Involves the mixing of one s orbital and three p orbitals, resulting in four sp3 hybrid orbitals. The bond angle is 109.5°, forming a tetrahedral geometry.
Example of Hybridization
Consider methane (CH4), where carbon undergoes sp3 hybridization to form four equivalent sp3 hybrid orbitals, each overlapping with a hydrogen atom’s 1s orbital to form four σ bonds.
Molecular Orbital Theory (MOT)
Molecular Orbital Theory provides a more advanced understanding of chemical bonding compared to VBT. It describes the molecular structure by considering electrons in molecular orbitals that are formed by the linear combination of atomic orbitals (LCAO).
Linear Combination of Atomic Orbitals (LCAO)
In LCAO, atomic orbitals combine to form molecular orbitals that are either bonding, antibonding, or non-bonding:
- Bonding Molecular Orbitals: Formed by the constructive interference of atomic orbitals, resulting in increased electron density between the nuclei.
- Antibonding Molecular Orbitals: Formed by the destructive interference of atomic orbitals, resulting in a node between the nuclei where the electron density is zero.
Types of Molecular Orbitals
Molecular orbitals are of two types:
- Sigma (σ) Molecular Orbitals: Formed by the end-to-end overlap of atomic orbitals along the internuclear axis.
- Pi (π) Molecular Orbitals: Formed by the side-to-side overlap of atomic orbitals perpendicular to the internuclear axis.
Electronic Configuration and Molecular Behavior
The filling of molecular orbitals follows the same principles as atomic orbitals, such as the Aufbau principle, Pauli exclusion principle, and Hund’s rule. The molecular electronic configuration helps determine the bond order, magnetic properties, and stability of the molecule.
Bonding in Homonuclear Diatomic Molecules
Homonuclear diatomic molecules are composed of two identical atoms, such as H<sub>2</sub>, O<sub>2</sub>, and N<sub>2</sub>. The molecular orbital theory effectively explains the bonding in these molecules by considering the combination of atomic orbitals from each atom to form bonding and antibonding molecular orbitals.
Example: Bonding in O<sub>2</sub>
In O<sub>2</sub>, the electronic configuration leads to the filling of both bonding and antibonding orbitals, resulting in a bond order of 2, which corresponds to a double bond between the oxygen atoms. This configuration also explains the paramagnetic nature of oxygen due to the presence of unpaired electrons in antibonding orbitals.
Hydrogen Bonding
Hydrogen bonding is a special type of dipole-dipole interaction between molecules. It occurs when a hydrogen atom bonded to a highly electronegative atom (such as N, O, or F) experiences an attraction to another electronegative atom in a different molecule or a different part of the same molecule.
Types of Hydrogen Bonds
- Intermolecular Hydrogen Bonding: Occurs between molecules, such as in water (H2O), where each water molecule can form hydrogen bonds with up to four other water molecules.
- Intramolecular Hydrogen Bonding: Occurs within a single molecule, such as in ortho-nitrophenol, where a hydrogen bond forms between the hydroxyl group and the nitro group within the same molecule.
Conclusion
This comprehensive guide on “Chemical Bonding and Molecular Structure” provides an in-depth exploration of the fundamental aspects of CBSE Class 11 Chemistry. It covers the core concepts of atomic bonding, the formation of molecules, and the structure of various compounds. By delving into topics such as Valence Bond Theory, Hybridization, and Molecular Orbital Theory, this guide helps students understand how atoms interact to form stable molecules. It also explains the different types of bonds, like sigma and pi bonds, and their significance in molecular geometry and behavior. Understanding these principles is crucial for grasping more advanced concepts in chemistry and for understanding the chemical processes that underlie biological systems.
This chapter lays the groundwork for studying the behavior of matter, both in isolation and in interaction with other substances, providing essential knowledge for future scientific endeavors. Explore more resources on the iPrep App to solidify your understanding of Chapter 4: Chemical Bonding and Molecular Structure with animated videos, practice questions, interactive quizzes, simulations, and more engaging content.
Practice questions on Chapter 4 - Chemical Bonding And Molecular Structure
Get your free Chapter 4 - Chemical Bonding And Molecular Structure practice quiz of 20+ questions & detailed solutions
Practice Now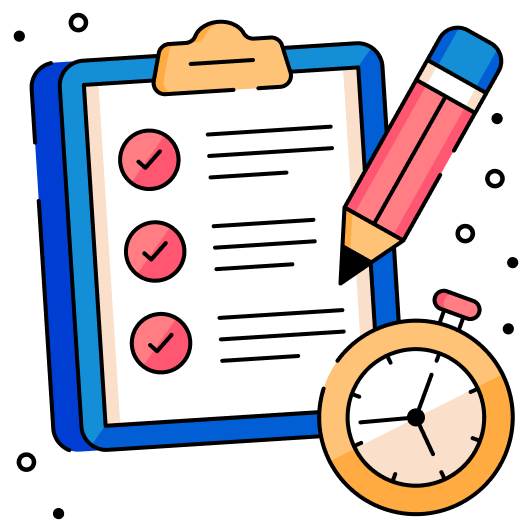